Thank you for visiting nature.com. You are using a browser version with limited support for CSS. To obtain the best experience, we recommend you use a more up to date browser (or turn off compatibility mode in Internet Explorer). In the meantime, to ensure continued support, we are displaying the site without styles and JavaScript.
Communications Materials volume 3, Article number: 64 (2022 ) Cite this article Nickel Alloys Ni307-7 Welding Data

Improved lithium batteries are in high demand for consumer electronics and electric vehicles. In order to accurately evaluate new materials and components, battery cells need to be fabricated and tested in a controlled environment. For the commonly used coin and small pouch cells, certain key factors and parameters substantially influence the final cell quality and performance. Therefore, to achieve accurate and reliable data on new materials for batteries, repeatability, and quality of cell fabrication are critical to ensure reproducible findings. Here, we discuss the key factors and parameters which influence cell fabrication and testing, including electrode uniformity, component dryness, electrode alignment, internal and external pressure, electrolyte amount control, and cell fixture with pressure control. We also provide general guidelines for reliable cell preparation.
Lithium-ion batteries (LIBs) were well recognized and applied in a wide variety of consumer electronic applications, such as mobile devices (e.g., computers, smart phones, mobile devices, etc.), power tools, as well as health maintaining devices1. Due to the emerged electrified transportation market request, the development of more advanced batteries with high energy density and low lost was also highly demanded2,3,4,5,6. Aside from studies and developments of traditional LIBs based on lithium (Li) intercalation between the graphite anode and lithium transition metal oxide cathode, Li metal battery system, in which metallic lithium anode is used against lithium metal oxide or other non-Li containing cathodes also drew much attention due to their potential with higher energy density or lower material cost comparing with traditional system5. As both Li-ion and Li-metal batteries utilize Li containing active materials and rely on redox chemistry associated with Li ion, we prefer the term of “lithium batteries” (LBs) to refer to both systems in the following context.
In recent decade, all major components of LBs, including active materials, binding agents, conductive additives, electrolyte, and membranes, were thoroughly investigated and documented by numerous amounts of published research results7,8,9,10,11,12,13. Besides material level chemical and physical characterization, electrochemical cells must be prepared and tested to further investigate the performance of those components. Ideally, commercially production line-made cell, no matter pouch, cylindrical, or prismatic format, provide better reproducible result6. However, most research labs still use small format cell, such as coin cell, for evaluation due to the resource and cost limitation14,15. Although some institutes use single-layer or small sized multi-layer pouch format cell for evaluation, most of those samples were still “manually-made” starting from active material powder (in contrast to auto- or semi auto- manufacture line-made). Until recently, research community started to pay attention to the reliability of cell fabrication, as the further evaluation is highly relied on the quality and consistency of the obtained cells. For coin format cell, several key factors have been identified along the whole cell fabrication process that have much influence on the final cell performance14,15,16,17,18,19,20. Unfortunately, studies on key factors for pouch format cell preparation and evaluation is still rare21.
In this article, key factors that affect the final cell performance along the fabrication process are identified and discussed for coin format and pouch format, respectively. Some important parameters which have significant influence on those factors are also introduced and discussed. At the end, some expectations on a systematic study on the cell fabrication process, as well as the need of standard protocol for cell making and testing are also presented. We wish the discussion on those key factors and important parameters would provide a general guideline on reliable and reproducible cell fabrication and testing to the battery research community.
Electrodes are the most important component in the LB cell. The quality of the electrode would largely affect the final cell performance. Back in 2011, Mark et al published a general method for LIBs electrode preparation using NMC111 cathode as an example, which is a reference for PVDF binder application18. In a recent publication, Jiangtao et al. provided an example of graphite anode preparation, which uses aqueous based carboxymethyl cellulose binder15. (Fig. 1.) In both examples, good mixing of the slurry was emphasized with appropriate mixing equipment setup and time control, as the uniformity of the slurry is one key factor that would influence the final cell performance. In order to reduce agglomeration, pre-grinding and sieving of solid powders (active materials and conductive additives) are highly recommended before wet mixing with binder solution. In addition, solid content should be consistent among different batches for better quality control.
a Slurry mixing step; b Cooling of slurry after mixing; c porosity control in calendaring process; d Electrode alignment during full cell fabrication; e N/P ratio control for full cell; f Electrolyte wetting on electrodes. (reprint with permission from ref. 15. Copyright 2021 Cell press).
The uniformity of the electrode thickness, especially with thick electrode coating, is a consequential critical factor to influence the final cell performance22,23. Each manufacturer has their own specifications and requirements on electrode coating thickness variation, which is usually within several percentage. However, most research labs still rely on hand tools or small motorized coating equipment to spread the slurry across the foil for small area coating. In order to achieve better coating quality, some parameters would be addressed for lab-scale coating operation. One of the important parameters is the coating speed. Depending on the viscosity of the slurry, the spreading speed may affect the final coating thickness even with the same gap setting. Considering the slurry feeding method to the coating blade, as well as the evaporation rate of the solvent, the coating thickness can have some variations at both beginning and ending area. However, a steady coating speed will provide relative uniform coating thickness along the coating direction. On the other hand, the gap of the coating blade should be carefully calibrated before use as it will affect the thickness variation along the coating width.
The dryness of all components such as electrolyte and separator membrane, is also critical towards cell performance. It is well known that off-controlled moisture content in batteries can result in unstable active material structure, gas evolution, as well as other safety issues8,24,25. Therefore, periodically checking the moisture content, as well as keeping the dryness of organic solvents and Li salts are necessary. For example, some ether-based solvents is highly hygroscopic due to the hydrogen-bonding formation with water. Even being stored within Ar glovebox, the moisture content will be increased, especially with frequent uses, less insulated container, and long storage time. In most cases, activated A4 molecular sieve would help keeping the dryness of the solvents with low initial moisture level (~10–20 ppm). Unlike organic solvent, Li salts need to be treated case by case. For example, LiTFSI can be re-dried under vacuum condition (e.g., drying on Schlenk-line), while LiPF6 does not have easy drying or recovering methods in general lab condition. For prepared electrolyte, even commercial electrolyte, checking the purity and moisture content periodically by Karl Fischer titration and NMR is highly recommended26,27, as A4 molecular sieve can not be used due to the ion-exchange.
The drying of separator before use is also highly recommended considering its highly porous nature. A common way for regular separator drying is using low temperature (e.g., <60 °C) vacuum process with controlled time to prevent the thermal deformation. In recent years, novel type separators that have new polymer components, or special surface coatings, have been introduced to battery area. For those new separators, use suggested drying condition from the manufacturer or vendor is highly recommended.
Coin cell parts, such as cathode and anode cases, spacers, and springs need to be carefully cleaned before drying. These metal parts, depending on the manufacturer process control, may have metal and organic residues. Acetone/alcohol and DI water rinse with ultrasonic bath assistance would help with removing those residues before further drying. Other cell component parts, such as pouch materials and tabs/tapes, should be also pre-dried before each batch cell making to prevent the accumulation of the moisture content.
Coin format cell is the dominant format used in battery study due to its simple configuration, easy preparation, and relatively low material cost. There are several key parameters have been identified that would affect the cell preparation quality and data repeatability14,15. The alignment of cathode and anode is very critical towards long cycling stability28. Ideally, the cathode and anode area should be the same with 100% overlapping14. However, this design always have electrode misalignment that lead to direct Li deposition thus resulting in inconsistent results. Therefore, the anode area should be slightly larger than cathode, which helps with better alignment15. This oversizing design is also used in commercial larger format cells. Another key factor is electrolyte amount used in coin cell assembling. In theory, electrolyte should fulfill all pores in electrodes and separator membrane. A systematic study on NMC/graphite full cells suggested appropriate excess of electrolyte brings better cell capacity20. To obtain better reproducibility of data from different experimental batches, exact same amount of electrolyte should be used in all cell preparations. On the other hand, using much excess electrolyte should be cautious as the total amount in each cell may varies, since the electrolyte may be “squeezed” out during crimping.
Besides above factors that were thoroughly studied, the pressure applied on the internal parts including electrodes and separators is also a critical factor that would affect the final cell performance. Figure 2 shows a cross-section diagram of typical coin cell. Unlike pouch format cell in which the applied pressure mainly from external source, the coin cell internal applied pressure on electrodes is mainly from the spring compression. Unfortunately, there was no reported study on the internal applied pressure. Variety of spring design and texture makes it difficult to obtain a baseline pressure profile. As the internal height of cell is fixed (e.g., ~3 mm for 2032 type coin cell), the spring compression is determined by the total thickness of other components, and selection of spacer thickness. Therefore, when use similar electrode thickness in different batches, same spacer thickness should be used in order to obtain a consistent spring compress, which links to the internal applied pressure. Meanwhile, when the electrode coating thickness or mass loading changes, or the Li counter electrode thickness changes, corresponding adjustment on spacer thickness is necessary to provide reasonable closed internal pressure condition.
Cross-section scheme of coin format cell with different internal pressures, assuming all components are the same except for different spacer thickness. a Higher internal pressure with bigger spring compression due to thicker spacer and resulting smaller spring gap; b Lower internal pressure with smaller spring compression due to thinner spacer and resulting bigger spring gap.
Besides the internal applied pressure, external pressure would be also applied by the crimping process. The crimping pressure varies from several hundred to thousand psi, depending on the equipment design and setup. Although the pressure reading doesn’t mean the final pressure applied on the internal component, it still affects the internal component with additional pressure during the crimping process. For example, too much-crimping pressure setup could induce the deformation of separator, thus resulting in the internal short. Unfortunately, it is hard to have a standard protocol for the crimping pressure setting, since the mechanics and designs of crimping tool varies. However, consistent setup including appropriate pressure setting and holding time could much reduce the cell-making failure rate and largely improve the coin cell data reproducibility.
As discussed in previous section, mostly manual-making process of the coin format cell has a big system deviation. For such systems, statistical analysis of data with enough sample cell numbers for each batch could be more appropriate and meaningful. Brandon et al. published a systematic study using 30-cell test20. The authors suggested smaller sample sets can be used to provide a reasonable spread in data. Since this area is more towards mathematical statistics which is beyond the scope of this article, we will not have further discussion. However, have enough sample set number for each batch (e.g., 3–10 cells per batch) should be encouraged in lab-scale research when using coin format cell.
In recent years, single-layer and small multi-layer (usually <3 Ah) pouch format cell were introduced in advanced LB research as they were considered more closed to their commercial counterpart than coin format cell21,29,30. However, as most of the lab-scale small pouch format cells were prepared manually, the quality and data reproducibility are also highly affected by all errors during the operation, similar to the coin format cell. A general pouch cell-making process includes electrode cutting/trimming, electrode stacking, tab welding, pouch sealing, electrolyte injection, formation, and final degassing and resealing. This pouch cell-making process contains more operation steps than coin cell-making, thus introducing more system and human errors.
Regardless of fabrication process, pouch format cell shares the similar key factors that link to the final cell performance. For example, the alignment of electrodes is still very critical no matter in single-layer or in multi-layer cells. In most lab-scale setup, the anode is about 1–2 mm bigger than cathode on each edge, therefore the tolerance for the misalignment is very small. Besides in initial placement of the electrodes, precautions on adjusting electrode alignment should be taken in following separator winding, tab welding, and pouch sealing processes. In the stacking process, the electrodes can be easily shifted during the electrode winding process due to static electricity. In the tab welding process, the misalignment would happen because of the tab area distortion due to the pressure from the welder heads. Similar pressure-induced misalignment would happen on pouch sealing as well, especially the edge with tabs. In most cases, some customized holder or jig which can apply some pressure and geometrically confines the jelly roll (electrode/separator stack) and cell would much help with the alignment.
Moreover, the electrolyte wetting time, especially for cells using thick coated electrode, should be controlled to allow complete diffusion of the electrolyte. In the battery industrial manufacture, the electrolyte injection and following wetting are carefully control by appropriate engineering design on both processing and equipment. Many critical factors are considered in the overall design, such as electrolyte viscosity, bulk diffusion rate, vapor pressure, and evaporation rate. In most research lab conditions, providing long enough soaking time with vacuum condition could be a more practical solution. Although there is no evidence supported by published data or result to suggest vacuum condition can help increasing the electrolyte wetting rate, a vacuum-sealed pouch can prevent electrolyte evaporation and external moisture/impurity during wetting process.
Electrolyte amount control is another factor for the overall cell performance. Pouch format cell has less “dead” space than coin format cell. However, how much “dead” or free space of pouch format cell is highly depending on the cell design. Generally, single-layer pouch cell always has more free space than multi-layer design (e.g., >5 layer), while small size cell (e.g., 0.5 Ah) has more free space than large size cell (e.g., 30 Ah). For research purpose, to get reproducible results on pouch format cell, electrolyte amount should be accurately controlled and measured. To obtain the accurate electrolyte amount, several separated measurements are necessary before and after the formation/resealing process, as the electrolyte will be consumed during the formation and removed partially with resealing of the pouch.
Dryness factor, which was generally discussed in above section needs to be re-emphasized for pouch format cell. Considering much larger surface area of electrode and separator, as well as much longer fabrication time than coin cell, pouch cell components may absorb more moisture and impurities during the fabrication, depending on the operation condition. Therefore, immediate usage of the pre-dry components and shorter operation time is highly recommended to reduce the impact from the moisture.
Unlike the coin format cell, the pressure applied on the internal components in pouch cell comes from the vacuum insides the pouch and external stacking pressure. Some previous reports suggested the external stacking pressure has influence on the cell impedance and current distribution, thus affecting the cycling performance in LIBs. For Li-metal battery, the influence of external stacking pressure is even more due to the pressure-sensitive nature of Li deposition condition31,32. Therefore, staking pressure which usually provided by cell fixture is another critical factor for the cell performance and data reproducibility. Most cell fixtures were customized with different designs for pressure control, as there are no commercially available products with universal design. As Fig. 3 shows, common cell fixtures either use two plates design with cell sandwiched in between or use three plates design with one “floating” board for better pressure distribution and control. Both designs use bolts located at corners to help fix the position of plates. In some cases, the pressure was controlled by a certain mass loading on the top plate. while the majority of these cell fixtures relies on the compression of springs on the bolts, which can be estimated by Hook’s law29. These cell fixtures with spring loading can work well on small-sized pouch cell (i.e., < 5 Ah). However, two parameters need to be pay attention to in making and using those fixtures – plate texture and spring compression calibration. Based on our experience, metals such as aluminum alloy or stainless-steel textures can provide better pressure distribution. In contrast, plastic or fiberglass textures are more flexible, which bends when applying high pressure on the corners. Besides plate texture, the springs should be also carefully selected and calibrated. If necessary, multi-point pressure calibration on different locations (as shown in Fig. 4) is recommended to achieve better pressure distribution in addition to spring compression control. In fact, in practical case, the spring compression by measuring the plate gap, or directly from spring length measurement, can’t provide accurate pressure determination due to the measurement error, spring quality, plate texture, and friction between the bolt and plate hole. Therefore, multi-point pressure check with flat-shaped pressure sensor will further help with the accuracy of the pressure control with external pressure supply, no matter spring type or load cells.
a Two-plate design with battery cell placed in the middle between plates; b Three-plate design with battery cell placed in the middle between two bottom plates.
a cross-section side view of the cell fixture and cell, with suggested calibration locations showed in red circles; b bottom view of the cell fixture and cell (bottom plate not shown for clear view) with suggested calibration locations showed in red circles.
When testing the LB cell, a dedicated thermal chamber with accurate temperature control is necessary to provide a stable testing environment. In early studies, many researchers use “room temperature” for the initial screening and study work. Considering the battery cycling is a long-term testing, the fluctuation of the environment temperature would affect the overall cell performance, especially with those temperature-sensitive systems using coin format cell which have fast heat exchange with environment. For pouch format cell, the heat exchange is slower with cell fixtures. Therefore, a certain resting time within the thermal chamber is necessary to stabilize the cell temperature.
Research community has realized the critical meaning of the reliable cell fabrication towards the valuable and reproducible battery study. As a good foundation of the whole battery research area, the cell fabrication should get more attention from the researchers. As we discussed in this article, there are many factors that do highly impact the reproducibility of the cell fabrication. Some of them such as electrode coating thickness control, dryness of components, or electrode alignment, are fall in engineering control strategy in manufacturing definition. There are scientific and engineering meanings behind every parameter determination. However, those studies are time consumable, experience/facility dependent, and not always aligned with academic research and study interests. Therefore, systematic investigation on those key parameters that affect the cell quality and performance is still rare, comparing with numerous publications on material or electrochemistry studies in the battery research area.
On one hand, we hope more research teams can get engaged in investigation and studying in this area to help provide solid evidence and analysis that would benefit the whole battery research community. One the other hand, we also hope this article can arise researchers’ attention to these factors and to further understand the physical and chemical parameters that link to the factors, thus, finding appropriate process, method, or solution to achieve reproducible and reliable results, based on the working condition and facility capability. Some of those factors, can be linked to certain physical parameters that can be measured and monitored. For example, the uniformity of the electrode control can be linked to viscosity of the slurry and particle size distribution, which can be measured by viscometer/rheometer, and particle analyzer, respectively. In electrolyte preparation, water content should be always tracked either by Karl-Fisher titration or NMR. For those physical parameters, researchers can pick appropriate lab-scaled characterization equipment and tools. Here we even suggest researchers add those characterization data to their publication, which not only provide better understanding of material property and process details, but also provide reference for further study.
Besides, when researchers investigate novel materials or components, selection of appropriate cell format and cell design that targeting different applications should also be taken into consideration. To eliminate errors that may brought by certain process or components is also an effective way to improve reproducibility and reliability. For example, in some study, single-layer pouch format cell with very small electrode area may not show better data consistency than a group of coin format cell with error analysis, due to its more complicated fabrication process, as well as difficult pressure control.
In order to further improve the cell fabrication reproducibility in the battery study, research groups and institutes should try to involve more auto- or semi auto- equipment in the cell fabrication process to largely eliminate the system errors by manual operation process. On the other hand, it could be a question and challenge for equipment manufacturers to make the appropriate lab-scale instruments to meet both technical requirement and budget limit. Besides, in some study, using electrode from commercial source, or any capable facility (e.g., large quantity electrode coating, pilot level equipment and facility) can help reduce much effort while achieve better consistency in electrolyte, separator, or special surface coating screening and evaluation, comparing with manually making the electrode in-house.
In this perspective article, we discussed some key factors in LBs cell preparation and evaluation. We hope this article can help not only draw attention from the battery research community on the impact of those factors and parameters on the final result reproducibility and reliability but also provide some initial solution and thought to address the questions. Aside from the extended study efforts, we also hope there will be further discussions on comprehensive cell preparation and evaluation protocols to provide operation standard and comparison baseline for future battery research and development. All these efforts can help bridge the gap between fundamental study and practical application of the LBs technology.
Goodenough, J. B. & Kim, Y. Challenges for rechargeable Li batteries. Chem. Mater. 22, 587–603 (2010).
Advances in Battery Technologies for Electric Vehicles. (Elsevier, 2015). https://doi.org/10.1016/C2014-0-02665-2.
Andre, D., Hain, H., Lamp, P., Maglia, F. & Stiaszny, B. Future high-energy density anode materials from an automotive application perspective. J. Mater. Chem. A 5, 17174–17198 (2017).
Chen, S., Dai, F. & Cai, M. Opportunities and challenges of high-energy lithium metal batteries for electric vehicle applications. ACS Energy Lett. 5, 3140–3151 (2020).
Schmuch, R., Wagner, R., Hörpel, G., Placke, T. & Winter, M. Performance and cost of materials for lithium-based rechargeable automotive batteries. Nat. Energy 3, 267–278 (2018).
Lensch-Franzen, C., Gohl, M., Schmalz, M. & Doguer, T. From cell to battery system - different cell formats and their system integration. MTZ Worldw. 81, 68–73 (2020).
Whittingham, M. S. Lithium batteries and cathode materials. Chem. Rev. 104, 4271–4301 (2004).
Xu, K. Nonaqueous liquid electrolytes for lithium-based rechargeable batteries. Chem. Rev. 104, 4303–4417 (2004).
Arora, P. & Zhang, Z. Battery separators. Chem. Rev. 104, 4419–4462 (2004).
Yang, Y., Zheng, G. & Cui, Y. Nanostructured sulfur cathodes. Chem. Soc. Rev. 42, 3018–3032 (2013).
Xiang, Y. et al. Advanced separators for lithium-ion and lithium-sulfur batteries: a review of recent progress. ChemSusChem 9, 3023–3039 (2016).
Etacheri, V., Marom, R., Elazari, R., Salitra, G. & Aurbach, D. Challenges in the development of advanced Li-ion batteries: A review. Energy Environ. Sci. 4, 3243–3262 (2011).
Lee, H., Yanilmaz, M., Toprakci, O., Fu, K. & Zhang, X. A review of recent developments in membrane separators for rechargeable lithium-ion batteries. Energy Environ. Sci. 7, 3857–3886 (2014).
Murray, V., Hall, D. S. & Dahn, J. R. A guide to full coin cell making for academic researchers. J. Electrochem. Soc. 166, A329–A333 (2019).
Hu, J. et al. Achieving highly reproducible results in graphite-based Li-ion full coin cells. Joule 5, 1011–1015 (2021).
Ruiz, V. Standards for the Performance and Durability Assessment of Electric Vehicle Batteries - Possible Performance Criteria for an Ecodesign Regulation. (2018). https://doi.org/10.2760/24743.
Zheng, G. et al. Amphiphilic surface modification of hollow carbon nanofibers for improved cycle life of lithium-sulfur batteries. Nano Lett. 13, 1265–1270 (2013).
Marks, T., Trussler, S., Smith, A. J., Xiong, D. & Dahn, J. R. A guide to Li-ion coin-cell electrode making for academic researchers. J. Electrochem. Soc. 158, A51 (2011).
Chen, S. et al. Critical parameters for evaluating coin cells and pouch cells of rechargeable Li-metal batteries. Joule 3, 1094–1105 (2019).
Long, B. R. et al. Enabling high-energy, high-voltage lithium-ion cells: standardization of coin-cell assembly, electrochemical testing, and evaluation of full cells. J. Electrochem. Soc. 163, A2999–A3009 (2016).
Müller, V. et al. Effects of mechanical compression on the aging and the expansion behavior of Si/C-Composite|NMC811 in different lithium-ion battery cell formats. J. Electrochem. Soc. 166, A3796–A3805 (2019).
Kim, H. et al. Failure mode of thick cathodes for Li-ion batteries: Variation of state-of-charge along the electrode thickness direction. Electrochim. Acta. 370, 137743 (2021).
Zheng, H., Li, J., Song, X., Liu, G. & Battaglia, V. S. A comprehensive understanding of electrode thickness effects on the electrochemical performances of Li-ion battery cathodes. Electrochim. Acta. 71, 258–265 (2012).
Yang, D., Li, X., Wu, N. & Tian, W. Effect of moisture content on the electrochemical performance of LiNi 1/3 Co 1/3 Mn 1/3 O 2 /graphite battery. Electrochim. Acta 188, 611–618 (2016).
Andersson, A. M. et al. Surface characterization of electrodes from high power lithium-ion batteries. J. Electrochem. Soc. 149, A1358 (2002).
Meyer, A. S. & Boyd, C. M. Determination of water by titration with coulometrically generated Karl Fischer reagent. Anal. Chem. 31, 215–219 (1959).
Schweiger, H.-G. et al. NMR determination of trace water in lithium salts for battery electrolytes. J. Electrochem. Soc. 152, A622 (2005).
Son, B. et al. Effect of cathode/anode area ratio on electrochemical performance of lithium-ion batteries. J. Power Sources 243, 641–647 (2013).
Mussa, A. S., Klett, M., Lindbergh, G. & Lindström, R. W. Effects of external pressure on the performance and ageing of single-layer lithium-ion pouch cells. J. Power Sources 385, 18–26 (2018).
Salihoglu, O. & Demir-Cakan, R. Factors affecting the proper functioning of a 3Ah Li-S pouch cell. J. Electrochem. Soc. 164, A2948–A2955 (2017).
Li, S. et al. Developing high-performance lithium metal anode in liquid electrolytes: challenges and progress. Adv. Mater. 30, 1706375 (2018).
Fang, C. et al. Pressure-tailored lithium deposition and dissolution in lithium metal batteries. Nat. Energy 6, 987–994 (2021).
General Motors Research and Development Center, 30470 Harley Earl Blvd, Warren, MI, 48092, USA
Fang Dai & Mei Cai
You can also search for this author in PubMed Google Scholar
You can also search for this author in PubMed Google Scholar
M.C. proposed the idea. F.D. and M.C. discussed and outlined the manuscript. F.D. conducted literature search and write the first draft. M.C. revised the draft. Both authors have given approval to the final version of the manuscript.
The authors declare no competing interests.
Communications Materials thanks the anonymous reviewers for their contribution to the peer review of this work. Primary Handling Editors: Jie Xiao and John Plummer. Peer reviewer reports are available.
Publisher’s note Springer Nature remains neutral with regard to jurisdictional claims in published maps and institutional affiliations.
Open Access This article is licensed under a Creative Commons Attribution 4.0 International License, which permits use, sharing, adaptation, distribution and reproduction in any medium or format, as long as you give appropriate credit to the original author(s) and the source, provide a link to the Creative Commons license, and indicate if changes were made. The images or other third party material in this article are included in the article’s Creative Commons license, unless indicated otherwise in a credit line to the material. If material is not included in the article’s Creative Commons license and your intended use is not permitted by statutory regulation or exceeds the permitted use, you will need to obtain permission directly from the copyright holder. To view a copy of this license, visit http://creativecommons.org/licenses/by/4.0/.
Dai, F., Cai, M. Best practices in lithium battery cell preparation and evaluation. Commun Mater 3, 64 (2022). https://doi.org/10.1038/s43246-022-00286-8
DOI: https://doi.org/10.1038/s43246-022-00286-8
Anyone you share the following link with will be able to read this content:
Sorry, a shareable link is not currently available for this article.
Provided by the Springer Nature SharedIt content-sharing initiative
The real story behind the research, from conception to publication, the highs and the lows
Communications Materials (Commun Mater) ISSN 2662-4443 (online)
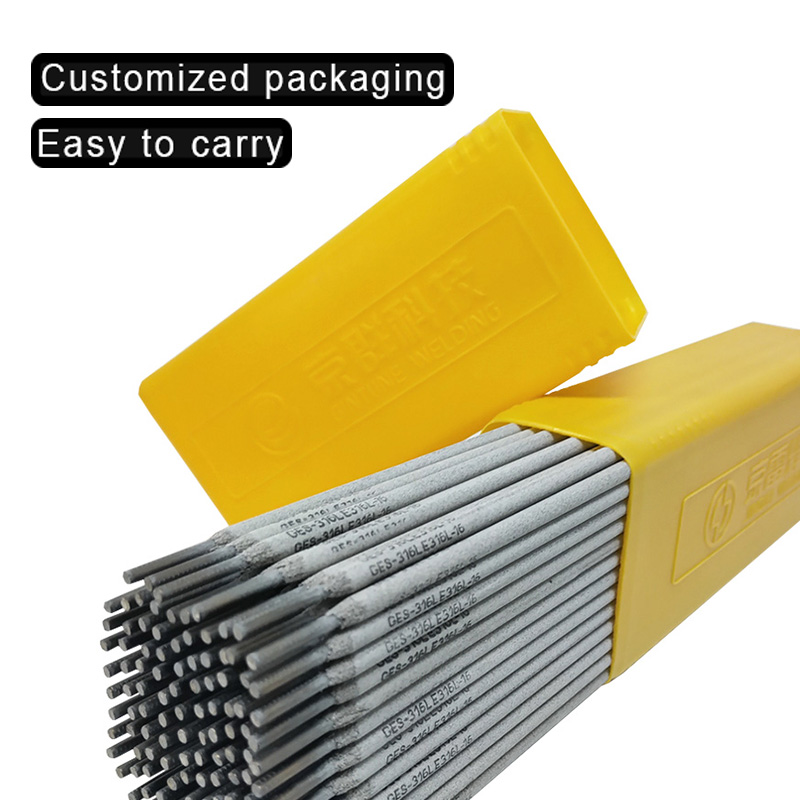
Welding Jointing Sign up for the Nature Briefing newsletter — what matters in science, free to your inbox daily.